The nanoscopes setting the stage for AI in biology
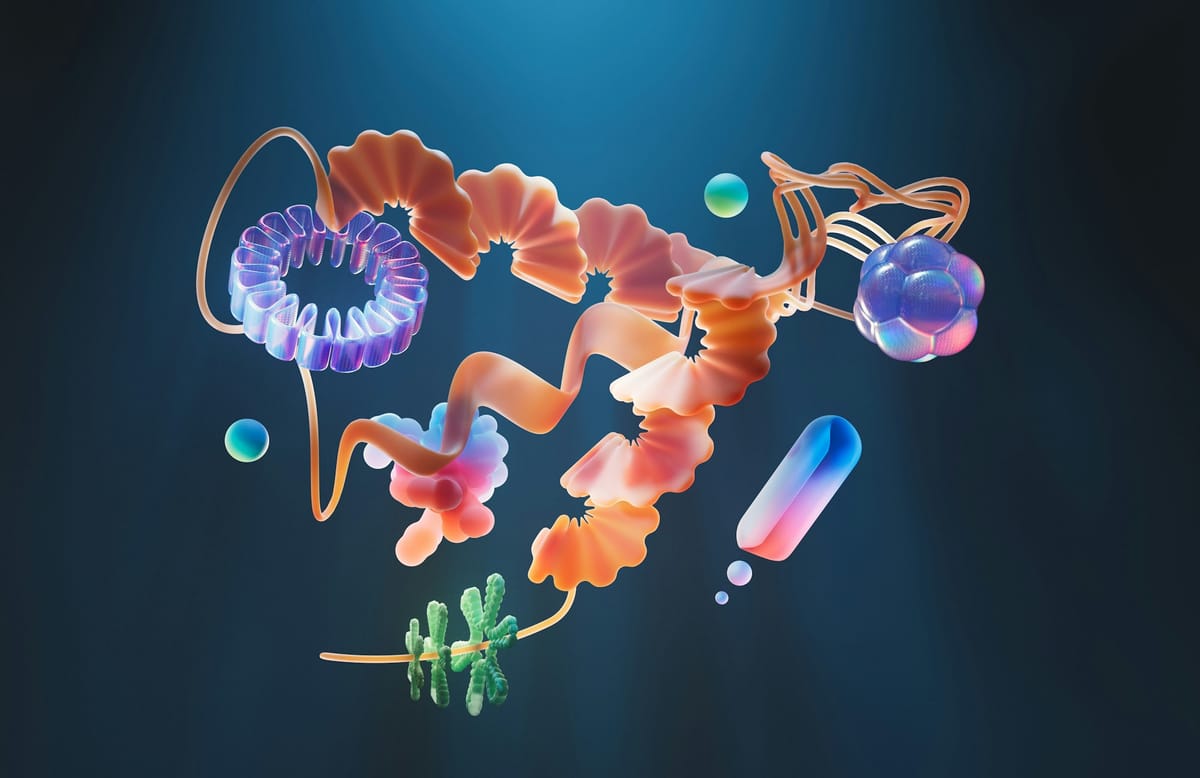
Förster resonance energy transfer (FRET) is a phenomenon in which one molecule transfers electronic energy to another molecule located some distance away. The transfer happens via the small electric field associated with the first molecule interacting with the field of the second.
FRET microscopy takes advantage of this phenomenon to observe objects smaller than 10 nm. When molecules called fluorophores are hit with electromagnetic radiation, they absorb it and glow with light of a lower frequency (including visible light); the latter is called fluorescence. Some fluorophores can be placed in a molecular setting and irradiated. FRET microscopy then estimates where other fluorophores are located, and thus where the parts of the molecule holding them are located, based on how the known fluorophores light them up through FRET.
For the last half century or so, FRET microscopy has been the optical technique of choice to understand how close one molecule is to another and to observe intramolecular interactions. But this may change now with a new method reported by researchers at the Max Planck Institute for Multidisciplinary Sciences in Göttingen, in the journal Science.
For its usefulness, FRET microscopy has many important limitations. Chief among them is that it’s an indirect way to measure distances, based on two electric fields interacting with each other to cause secondary fluorescence. If the fields are oriented relative to each other in a less than ideal way or if an electric field from a different source gets in the way, the output quality is diminished.
The new method offers to get rid of these confounding factors and produce a more direct result. It’s based on MINFLUX (short for ‘minimal fluorescence photon flux microscopy’), a technique the same researchers had reported in 2016. FRET microscopy works better if more fluorophores light up, which means more light is required to first illuminate them.
MINFLUX goes the other way. It also begins by embedding fluorophores in a sample, then shines a small dose of UV light on it. This light beam is shaped like a donut, with zero intensity at the centre and increasing towards the outer edge. When the beam irradiates a probing area, the fluorophores at different locations light up to different extents based on the intensity of the light they receive. Based on this pattern, MINFLUX starts a second scan, this time tightening its beam to increase the odds of lighting up some fluorophores over others. The fluorophores are bound to molecules, so successive tightening basically zeroes in on individual molecules and the distances between them.

Because the tightening requires fewer fluorophores to light up each time, MINFLUX is more sensitive than FRET. According to Meta AI (subsequently verified on Wikipedia), “the Cramér-Rao limit* provides a fundamental limit on the accuracy of parameter estimation”. For MINFLUX, this means its precision limit is directly proportional to the width of the scanning beam and inversely proportional to the square root of the fluorescent light’s intensity. So the wider the beam and the less the fluorophores light up, the better MINFLUX will perform.
Indeed, with the right equipment and amount of computing resources, the beam can be moved just a few nanometres at a time and locate a molecule within around five microseconds (i.e., five-millionths of a second).
Aside: The ‘Rao’ in the Cramér-Rao limit is CR Rao, the rockstar statistician from Bellary whose work influenced fields ranging from risk analysis to quantum physics. He passed away in August 2023. The Cramér-Rao bound arose in a paper Rao published in 1945 entitled ‘Information and accuracy attainable in the estimation of statistical parameters’, when he was only 25. Another result from the same work became the Rao-Blackwell theorem.
The new method reported in Science upgrades MINFLUX using better fluorophores. Fluorophores are molecules, too, and if they’re too close to each other, they could interact with each other. Microscopy methods based on fluorophores assume they operate independently of each other and respond only to the irradiating light. MINFLUX challenges this assumption by expecting the fluorophores to work independently even when they’re less than 10 nm of each other — and this may not always be the case. The researchers therefore used a fluorophore that would be chemically inert around others of its kind and respond only to the excitation beam.
When they tested their idea, the researchers found their version of MINFLUX could measure distances as small as 0.1 nm — about the width of a single phosphorus atom — and as large as 12 nm, an unusually wide range for a microscope operating at this scale.
MINFLUX is an example of super-resolution microscopy, the label for methods that use light while managing to achieve resolutions beyond the diffraction limit. In the late 19th century, German engineer Ernst Karl Abbe reported that the smallest distance a microscope can resolve is limited to the wavelength of the irradiating light divided by twice the numerical aperture. As a result, optical microscopes were limited for a long time to resolving distances no less than 2 micrometres.
From the late 1980s, scientists began to develop the new techniques that would become super-resolution microscopy, work for which Eric Betzig, Stefan Hell, and William Moerner were awarded the chemistry Nobel Prize in 2014. This trio (plus many others of course) beat the diffraction limit using fluorescence microscopy.
One of its earliest versions was stimulated emission depletion microscopy, conceived by Hell. A swath of fluorescent molecules was lit up in a sample before a second beam allowed the light from all molecules except those in a small patch at the centre to fade out — somewhat like collecting a large mass of water and draining all but the fish. (The draining allows the microscope to sidestep the diffraction limit.) Hell is also one of the authors of the new study.
Other super-resolution microscopy methods include PALM/STORM and PAINT. PALM, co-developed by Bertzig, reconstructs a large molecule’s shape by progressively activating a small number of fluorophores in random patterns until all of them have fluoresced. STORM is PALM with different fluorophores. PAINT localises molecules by allowing fluorophores to randomly attach with and detach from them, producing blinks of light that guide a computer to the molecules. All these methods, including MINFLUX, are supplemented with bespoke single-molecule localisation microscopy algorithms.
That chemistry Nobel Prizes recognised the advent of super-resolution microscopy and AlphaFold 2 precisely 10 years apart may be no more than a reflection of our choice of a number system (as Kip Thorne quipped here) but the two enterprises are closely, inextricably linked. The new AI-based tools to predict the structure of proteins reinforce the importance of the microscopy techniques, both to generate better data with which to train AI models and to verify the models’ predictions with real-world data.